Posted on American Museum of Natural History: This profile was developed for Week 3 of the AMNH online course Climate Change, part of Seminars on Science, a program of online graduate-level professional development courses for K-12 educators
Whether they work in the field or in the lab, many marine biologists study species in their present habitats. But Gretchen Hofmann looks to the future. She’s developed a way to simulate oceans affected by global warming in order to understand how ocean acidification may affect sea urchins in the years to come.
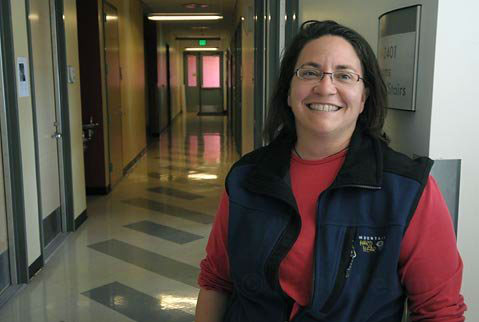
The effects of ocean acidification on tiny marine creatures such as the pteropod (seen in the inset) is a research specialty of marine biologist Gretchen Hofmann at the University of California at Santa Barbara.
Hofmann proudly describes herself as a “second-generation climate scientist.” Her physicist father studied the ozone hole in the Antarctic and also directed NOAA’s climate modeling group, so her childhood was steeped in atmospheric carbon dioxide levels and the Keeling Curve-not the usual dinner-table conversation. This background, combined with her strong interest in how animals interact with their environment, led her to marine biology in the context of climate change. “I was attracted to the ocean because of those two colliding interests,” she explains.
Ecological Physiology: Studying Species in Their Environments
Currently a professor at the University of California at Santa Barbara, Hofmann’s work focuses on the ecological physiology of marine organisms, especially invertebrates, perciform fishes (bony fishes that make up the largest group in the world), and kelp.
Physiology is the study of how plants and animals function on a biological level, and ecological physiology looks at how they function within their specific environments. In other words, Hofmann and her colleagues study how and why species live where they live-how their range and performance are determined by environmental conditions, as well as by other factors such as predators and food supply. For example, temperature can have a large impact on how the cardiovascular system functions in fish. “Maybe you have an Antarctic fish that lives in these incredibly cold temperatures, almost -2°C, just above the freezing point of seawater. Yet very similar species live in New Zealand, and they swim around in water that is 10°C.” So Hofmann asks functional questions in an ecological context: “How do fish swim, and how fast can they swim, and what’s the energy cost of swimming in these different conditions?”
How Do Species Respond to Environmental Change?
Beyond looking at differences between environments, ecological physiology also considers the impact of changes within environments, such as those caused by climate change. When an environment changes, there are four possible ways for species to respond. First, the species could migrate. While the ability to migrate can benefit the species at risk, it can be bad news for other species that depend upon it, including humans. Suppose, for example, that the species comprises an important fishery. “If there are no more salmon in Alaska, that’s really bad ecologically, and economically for humans,” Hofmann elaborates. And migration is not always an option, as in the case of polar bears. “If you are a cold-adapted organism and you are used to Arctic temperatures, where the heck do you go?” asks Hofmann. “Some polar biologists think that those regions are very much in danger of high rates of extinction. If you want a poster child for climate change, the polar bear would be it.”
The second possibility is that the species might be able to tolerate the environmental changes. “This means that the species somehow has some flexibility, that the group can tolerate the changing conditions around it,” Hofmann says. “That’s good news, because maybe you have some changes in how many of the organisms are there, but basically they don’t disappear.”
“The third one is true adaptation,” Hofmann continues, “where you have evolution operating to change the way an organism works. As we understand it, that process takes a long time-thousands, if not tens and hundreds of thousands, of years. So we are not sure if rapid evolution is going to be a viable solution for some organisms in the face of climate change.”
And the fourth possibility is extinction. Without the ability to migrate, tolerate changing conditions, or quickly adapt, a species dies out.
Hofmann’s research focuses on the second possibility-the ability of species to tolerate environmental change. Given the unusually rapid and potentially extensive changes caused by global warming, this is a vitally important research area. “We are asking about species vulnerability,” Hofmann explains. “If we know that conditions are going to change pretty quickly, probably more rapidly than evolution can operate on, what organisms out there have the physiological plasticity, the flexibility, to tolerate these new conditions?”
It’s Not Just the Temperature: The Second CO2 Problem
The “first” CO2 problem, of course, is the role of CO2 as a “greenhouse gas”: CO2 produced by fossil-fuel burning accumulates in the atmosphere and contributes to global warming. Oceanographers have long known that the ocean absorbs carbon dioxide from the atmosphere, a natural process that is part of the larger planetary carbon cycle. The absorption of excess CO2, says Hofmann, was initially seen as “a service that the ocean was performing for us. Then people began to realize that, in fact, all the CO2 being absorbed by the ocean was also altering the pH and the chemical composition of the ocean.” Hence the “second” CO2 problem.
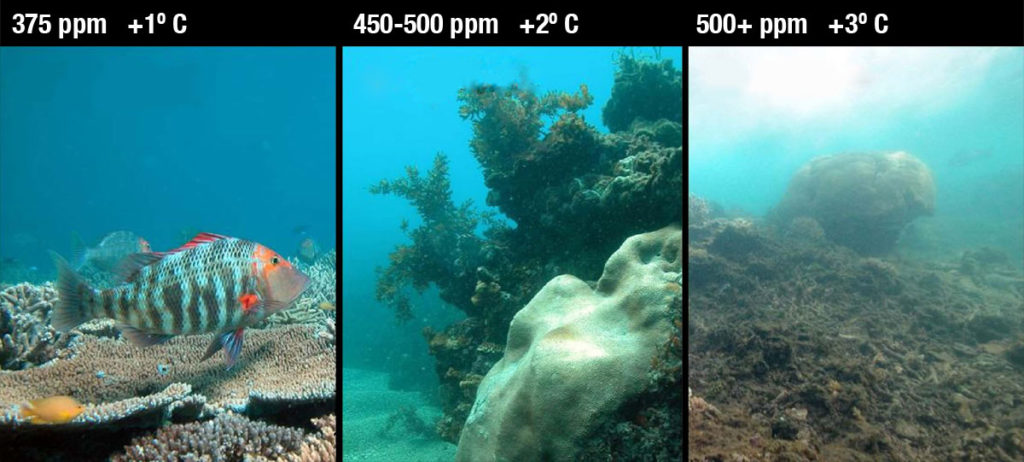
Increasing concentrations of atmospheric carbon dioxide are warming and acidifying the oceans. Effects of increasing carbon dioxide and increasing temperature on coral reefs can be seen in the three different ecosystems above. Warming and acidification will have devastating impacts on marine biodiversity and human livelihoods, especially in developing nations that depend on reefs for much of their economic well-being. ©NOAA
It was biologists studying coral reef systems in the 1990s who first realized that changes in ocean chemistry could affect, and even threaten, the process by which corals make their calcium carbonate structures. As a result, the scientific community began taking regular measurements of ocean chemistry. Several places around the globe-Bermuda, Hawaii, the Canary Islands-now have records of ocean chemistry from about 1995 forward. Like a Keeling Curve of the oceans, “these three long-term data sets are showing that the CO2 of surface ocean waters at these locations is going up, and pH is going down,” Hofmann says. “You could put those figures side by side and say, O.K., here is what’s going on in the atmosphere over Hawaii, and here is what is happening at these ocean sites, and they’re moving in concert, unfortunately.”
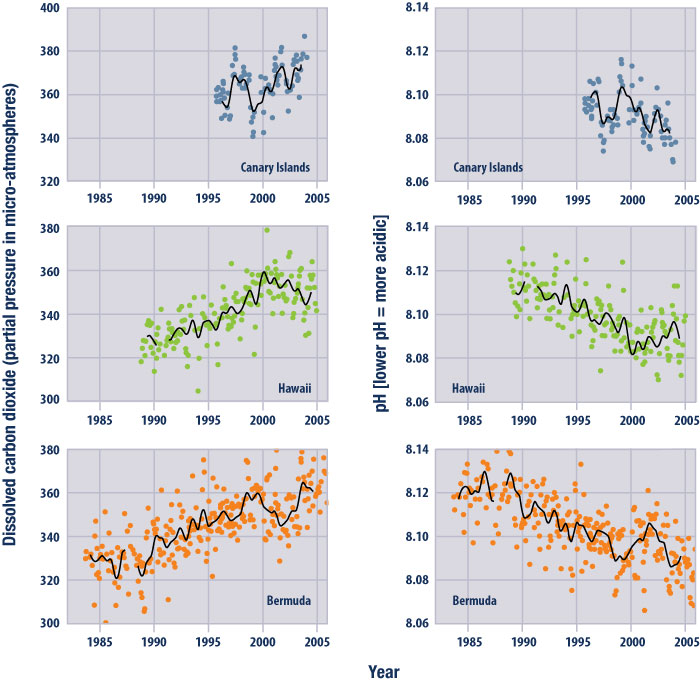
This figure shows changes in ocean carbon dioxide levels (measured as partial pressure) and acidity (measured as pH). The data came from two observation stations in the North Atlantic Ocean (Canary Islands and Bermuda; Bindoff et al., 2007) and one in the Pacific (Hawaii). Dots represent individual, while the lines represent smoothed trends. ©EPA
Carbon dioxide in seawater sets off a chain of reactions that alters the ocean’s pH, a measure of how acidic or basic the water is. The pH scale runs from 0 (highly acidic) to 14 (highly alkaline). For hard-shelled marine organisms (like clams, corals, and hermit crabs), water with a pH of about 8.2 is ideal. This chemistry makes it easy to assemble external skeletons from bicarbonate ions, the main form of carbon dissolved in the ocean. But the increase in carbon dioxide is decreasing the ocean’s pH dropping it to 8.05 on average. This change is hampering the development of these creatures, leaving them more vulnerable to environmental stressors. Although the ocean contains more carbon, in more acidic water less is available in the carbonate form these organisms require.
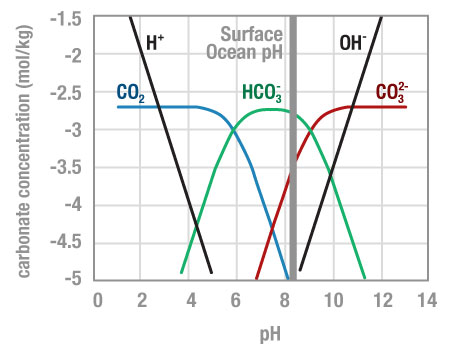
This graph shows typical concentrations of dissolved carbonate species in seawater as a function of pH. ©Richard Zeebe / EOE
So global warming leads to both temperature change and ocean acidification, which combine to create a kind of “double jeopardy.” “What does that mean for biology and the water?” asks Hofmann, who has become fascinated by the question. “And now we are talking about everything: microbes, phytoplankton, zooplankton … not just bigger animals like the sea urchins.” A new research study was born.
To investigate these questions, Hofmann studies sea urchins. Originally she focused on their ability to tolerate the temperature changes of global warming, but her research has since shifted to the impact of ocean acidification. Sea urchins are model organisms for studies of ocean acidification for four main reasons. First, sea urchins are important members of many marine ecosystems, so their health has cascading effects through entire communities, both natural and commercial. Second, urchins are distributed across tropical, temperate, and polar coasts worldwide, habitats that will be among the earliest affected by ocean acidification and the upwelling of deep corrosive waters. Third, to calcify their bodies, urchins use a form of calcium carbonate known as aragonite that is especially vulnerable to dissolution as pH decreases. And finally, because of the long history of sea urchins as model research organisms, extensive genomic resources are available. This enables scientists to explore the expression of genes involved in important biological processes such as biomineralization, growth, and metabolism.
The Life of a Stressed Sea Urchin
Hofmann had already been raising sea urchins in tanks in order to study the effects of temperature change. She has since shifted her focus from temperature to CO2 concentration. At her laboratory, Hofmann can “mix and bubble carbon dioxide gas” into multiple tanks of seawater to create simulations of the present-day ocean, at about 385 ppm CO2, with a pH of about 8.10. She can also create potential future oceans, such as seawater at 800 ppm CO2, which causes pH to decline to about 7.75. Hofmann then raises sea urchins from embryos to see how they develop and function in response to these potentially stressful environments.
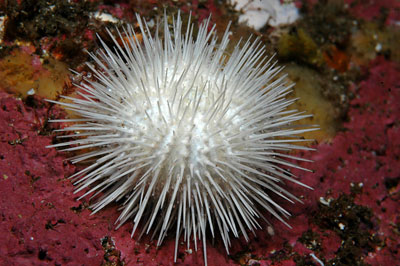
Sea urchins (like this example from New Zealand) are model organisms in that they are extensively studied to understand particular biological phenomena, with the expectation that discoveries made in the organism model will provide insight into the workings of other organisms. ©Ian Skipworth
So how do you measure stress in a sea urchin? “Stress in a cold-blooded, living-in-the-ocean kind of organism is something that threatens its physiological integrity,” Hofmann explains. “It messes up the way that it works.” To understand exactly how stressful low pHs levels can be, she “asks” a number of questions of her tanks full of sea urchin larvae, whose developing skeletons are visible under a microscope: “How are you doing in there? Are you building your skeleton? Are you making your body?” And as she asks those questions, she also wants to know the costs: “Are you having to use more of your energy to develop because it’s hard to deal with these low pHs?” To find out, Hofmann photographs them under a microscope and measures their skeletons.
“What we find,” Hofmann explains, “is that the larvae that develop in low pH or high CO2 as compared to standard conditions tend to be smaller at any given time. The CO2 causes a developmental delay. We can’t quite tell if they’re going to be smaller when they become adults. We’re still studying that. But definitely the CO2 has an impact on their growth rate.”
Hofmann also wondered whether lower pH levels would activate genetic defense mechanisms. “If you heat-stress embryos a little bit, they will turn on all these genes that produce proteins that then protect other parts of the cell and other proteins. It’s a classic defense mechanism in all cells,” she explains. Contrary to expectations, sea urchins do not respond to increased CO2 and lower pH as stressful on a genetic level. Instead, Hofmann’s research suggests that in response they experience a global metabolic depression. Genes that are important for maintaining protein integrity, controlling biomineralization and skeletogenesis, defending against oxidative stress, initiating the “fight or flight” response, and defending against toxicants, metals, and xenobiotics have all been seen to down-regulate under elevated CO2 conditions. These results suggest that even moderate acidification can impair the cellular stress-response pathway and diminish the capacity of urchins, particularly in the larval stage, to maintain their protective biological mechanisms. This means that growing up in a high CO2 world could greatly reduce the organism’s capacity to tolerate additional stressors such as warmer temperatures.
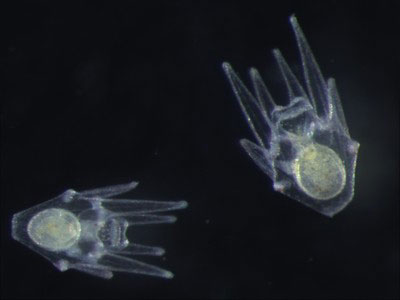
The deleterious impact of ocean acidification on the development and calcification process in embryos and larvae of the purple sea urchinStrongylocentrotus purpuratus, an ecologically critical marine invertebrate found along the Pacific coast of North America. ©UCSB
Most of this research takes place in the miniature oceans in Hofmann’s lab, but it’s also informed by research in the field. She travels widely to collect new specimens and set up logging devices to measure pH levels, and also relies on data from other environmental studies. Using her research on California sea urchin species as an example, she notes, “As we are able to collect more and more environmental data and get more observations, we’re learning that there might be more variation here on the California coast than we thought.” Because many modern populations of urchins (and other species) are already adapted to some degree of environmental pH variability-due to natural upwelling events, fluctuations in tidal cycles, and even daily respiration by kelp forests or other local biota-their potential tolerances for future acidification may be better than initially expected. “We need to do our lab experiments in a way that matches the environment,” Hofmann continues, “so that we make the appropriate predictions.”
The Larger Impact
The impact of ocean acidification goes far beyond individual species. Sea urchins, for example, occupy an important niche in marine ecosystems in intertidal zones along the coast of California and Oregon, as grazers who eat algae and kelp. If there are too many urchins, they can graze down kelp forests and destroy them, while too few might lead to too much algae in the ecosystem. In addition, the red sea urchin is one of the top-grossing catch-value fisheries in California, with the gonads sold as a kind of sushi called uni. “Most people don’t have uni for dinner. And most people don’t know that Japan buys 80% of our sea urchin crop, and it’s worth millions of dollars to people on the West Coast,” Hofmann points out. “This is the kind of concern that cattleman might have in the American West if grazing were threatened.”
Hofmann and her research group are also targeting other species of marine invertebrates that are important both ecologically and economically, including abalone and oysters. Ocean acidification has already had a devastating effect on oyster hatcheries in Oregon, in some cases killing off all the oyster larvae (spat). “These hatcheries are really important, because they supply spat and materials for oyster farmers to grow,” Hofmann says. “Here is an example where a climate-change-related phenomena, ocean acidification, is impacting one ecological community and one business area really heavily.”
Equally in danger are tropical reefs, highly biodiverse ecosystems that are made of the calcium carbonate skeletons of coral and can be large enough to be seen from space. “Not only do you lose the beautiful, colorful, hard coral,” Hofmann cautions, “but you lose the habitat for the fish that people are eating, and for all sorts of other organisms. The coral themselves are so important to that habitat and that environment that if we lose them the whole system crashes.”
While the scientific community has come to realize the great threat posed by ocean acidification, the general public is still mostly unaware. Hofmann thinks that this is probably due to the fact that the issue is far removed from most people’s lives. But as the impacts are felt more widely, greater awareness will follow.
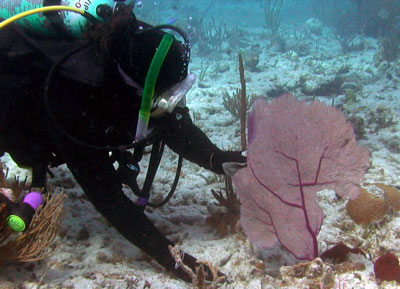
Although the research projects in Gretchen’s lab are quite diverse, Gretchen and her colleagues are collectively interested in understanding the role of temperature and oceanographic features in setting species’ distribution patterns in the marine environment. ©UCSB
What’s Next?
In the near future, Hofmann plans to bring together her early research into temperature with her current research on acidification, to explore how a “multi-stressor situation” might impact larval forms of marine invertebrates. Her preliminary research suggests that exposure to both higher temperature and ocean acidification does not simply lead to an additive outcome of the kind seen when species encounter these stressors in isolation. Depending on the species and the magnitude of the stresses involved, the physiological responses can be surprisingly complex. Therefore, in both the laboratory setting and natural environments, Hofmann plans to explore how adult and larval urchins alter their metabolism, energy usage, biomineralization, and gene expression in response to a “multi-stress” context.
She would also like to do more work in the Arctic and the Antarctic, regions that are among the first to be deeply affected by climate change. Cold water holds more dissolved gas, so cold oceans will have higher concentrations of CO2 earlier, and therefore will become more acidic first. But combining acidification with potentially warmer temperatures creates an apparent paradox: warmer waters will be less acidic, but may grow too warm for cold-adapted species to tolerate.”
Speaking as a citizen, Hofmann does believe that we can choose to change our behavior to reduce the impacts of climate change. But it may take a while. In the meantime, she wonders how many of the marine organisms that she studies “have the tolerance to hang on for the next hundred years while we figure this out.”
Related Links
USCB: Hofmann Laboratory
The website of the Hofmann Laboratory describes how Hofmann and her colleagues are investigating the ways in which marine organisms are evolving and adapting to a changing ocean.
http://hofmannlab.msi.ucsb.edu
Stanford: Virtual Urchin
Prepared by scientists at Stanford University’s Hopkins Marine Station, these 8 interactive tutorials enable teachers and students alike to observe sea urchin fertilization, cell division and embryonic development.
http://virtualurchin.stanford.edu
EPOCA: FAQs About Ocean Acidification
Frequently asked questions about ocean acidification, answered by the international climate-science research community. Topics range from why it’s called ocean acidification to the effects on various marine ecosystems and the role of policymakers.
http://www.epoca-project.eu/index.php/FAQ.html
This profile was developed for Week 3 of the AMNH online course Climate Change, part of Seminars on Science, a program of online graduate-level professional development courses for K-12 educators. Explore more sample resources…